Higgs Festival
Event description
The Higgs Centre is celebrating 10 years since Peter Higgs was awarded the Nobel Prize in Physics (in 2013), alongside François Englert, for the theory of how particles acquire mass.
Peter Higgs is an Edinburgh local and conducted his research at the University of Edinburgh.
From 14:00, continuing the whole afternoon
Meet theoretical physicists, attend interactive shows with the "Bad Boy of Science" Dr Sam Gregson, make your own crafty particle collision to take home, have a look at our art exhibition and visit our social media booth.
Interactive Show with Dr Sam Gregson at 15:00
The amazing discovery of the Higgs Boson in 2012 by CERN, and anticipated by Peter Higgs and François Englert (and R Brout) in the 1960s was one of the most important scientific discoveries of this century. But could you have made the discovery for yourself?
In this highly interactive, highly energetic, comedic show, you will take control of the infamous large hadron collider - the world's largest and most powerful atom smasher. Can you hunt the Higgs and claim the Nobel prize for yourself?
Movie Premiere at 17:00
Another highlight of the afternoon will be the exclusive first look at our short documentary film tracing the development of theoretical, mathematical and computational physics in Edinburgh (From Tait to Higgs) with a panel discussion on the accompanying historical research project, which was conducted by Mariana Duarte.
If you're planning to attend the science show and/or the movie premiere, please register (free) as there is limited capacity due to room size.
Resources
Fun Facts about Peter Higgs and the Higgs Boson.
An essay
Studying physics is a way to get an understanding of the Universe we live in. Particle physics aims at describing the Universe at the shortest length scales ever reached in experi- ments, the so-called microcosmos. Today we can reach scales on the order of 10−19 meters, i.e. ten billion times smaller than a nanometer, which in turn is one billion times smaller than the height of an average human. The world works fundamentally different in this setting, in particular as it is inherently quantum mechanical, and our goal as particle physicists is to describe how the elementary particles in the microcosmos interact with each other. The best theoretical knowledge we have of these fundamental building blocks of our Universe is contained in the Standard Model of particle physics.The Standard Model contains 17 particles that all have been observed in experiments. Six of these are quarks, six are leptons and four mediate the electromagnetic, strong and weak nuclear forces. The electromagnetic force describes how charged particles affect each other, the strong force binds together quarks into atoms which constitute all matter we see around us, and the weak nuclear force powers our sun. The attentive reader has probably already seen that I so far only enumerated 16 particles out of the 17 in the Standard Model. The remaining one is the so-called ‘Higgs boson’, which was hypothesised in 1964 and was only experimentally discovered at the Large Hadron Collider in Switzerland in 2012. The discovery subsequently led to the Nobel Prize in physics the following year.
So why is the Higgs boson so important? The original answer to this question comes from the masses of the particles we can observe. If we conduct a particle physics experiment where we collide e.g. protons at very high energy, we can from Einstein’s theory of relativity create new particles that can be observed in the experiment. Almost all particles in the Standard Model have mass, in particular the quarks and leptons building up the matter we see around us. Before we knew about the Higgs boson and the associated so-called Higgs mechanism, we did not understand the origin of mass. This is clearly very alarming to particle physicists who would like to fully understand the inner workings of the microcosmos. The conceptual leap taken in 1964 by introducing a new particle that in an elegant way can explain why the particles we observe have masses is truly an amazing thing to do, especially now in hindsight as the Higgs boson has been experimentally observed. This was the motivation given by the Nobel Prize committee in Sweden in 2013.
Even though it here might sound like the Higgs boson only exists to give other particles mass, this is far from the truth. This final cornerstone of the Standard Model in fact has many implications also for other phenomena in particle physics, and has itself also spurred new questions. One new question that immediately came up with the discovery of the Higgs boson is why it is so light. Its mass is 125 GeV/c2, where GeV is an energy unit and c is the speed of light which through Einstein’s relation E = mc2 shows that GeV/c2 indeed is a unit for mass. The value on its own perhaps might not say very much to non-particle physicists, but it is for various reasons very light from the point of view of the quantum theory we call the Standard Model. The seemingly unnatural fine-tuning of the light Higgs boson mass has caused much distress for particle physicists, and has led to ideas about other unknown particles we have yet to observe.
Apart from this esoteric conundrum on the lightness of the mass, the Higgs boson can also be related to bigger questions we can ask ourselves by just looking at what we see around us. In the Standard Model, every particle has a corresponding antiparticle, which annihilate into energy whenever they meet. If we look around us, everything we see is made of normal matter, as opposed to antimatter. This is very mind-boggling if we assume that the Universe had equal amounts of matter and antimatter at the beginning of time, which is a valid assumption given our knowledge and observations in particle physics experiments. It turns out that the Higgs mechanism can be seen as a phase transition in the early Universe, where bubbles of a new Higgs phase where particles acquire mass are formed (much like the bubbles of steam created in a pot of boiling water). From some additional rather intricate reasoning it is then possible to show that an asymmetry between matter and antimatter can be generated in a Universe that was created with equal amounts. It turns out that the Standard Model does not quite seem to be able to produce the asymmetry we observe in the Universe today, so there are again hints that there might be new particles that we must search for in experiments.
To conclude, the Higgs boson was proposed to answer questions that were at the minds of particle physicists in the early 1960s. Roughly 50 years later, the particle was discovered and resulted in a Nobel Prize. Even more importantly, the Higgs boson inspired a plethora of new questions that in the future will continue to guide us on our way to improve our knowledge of the Universe even further.
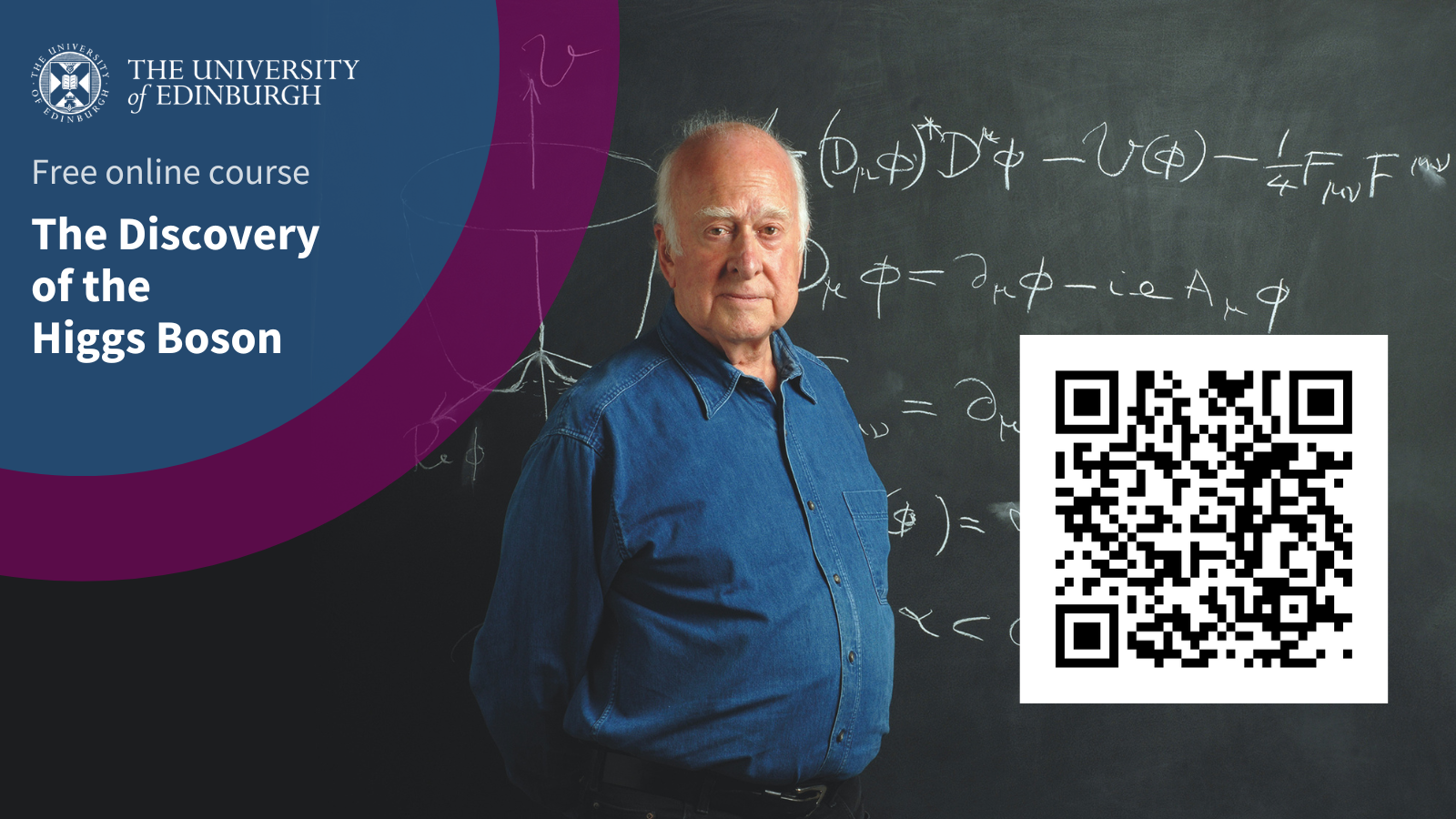
This (free online)[https://edin.ac/3FoXXzC] course introduces the theoretical tools needed to appreciate the discovery, and presents the elementary particles that have been discovered at the tiniest scales ever explored. Beginning with basic concepts in classical mechanics, the story unfolds through relativity and quantum mechanics, describing forces, matter and the unification of theories with an understanding driven by the tools of mathematics.
Higgs Festival
Venue
Thomas Bayes Rd
Edinburgh
EH9 3FG
Related events
This event is part of:
Contacts
- Ines Foidl(
- University of Edinburgh
Online
Password: higgs2013 (only the movie portion of the programme at 5pm will be available to watch online).
Find us on social media:
TwitterFacebookYouTube